Project
This proposal is an attempt to explore the fascinating challenges in the area with two broad categories of molecular solids, namely metal clusters and gas hydrates. Important strides have already been made in the study of molecular forms consisting of atomically precise metal clusters containing tens to hundreds of atoms; the participating groups have been among the leaders in this area. The challenge now is to fill in the ‘gap’ between molecules and bulk materials, by achieving clusters of hundreds of kilodaltons and eventually megadaltons of mass. Chemically and thermally stable atomically precise building blocks allow them to be assembled at will to create cluster co-crystals having multiple properties such as magnetism and luminescence, or chirality and luminescence, which unveils new properties. The variety and diversity available are numerous and opens new challenges. Moreover, stable clusters in all the three phases will allow their phase diagrams to be explored. Commercially useful cluster materials are likely.
In gas hydrates, our principal effort will be to use the hydrate cages new molecular containers in which cryogenic chemistry can occur. One of the pertinent questions in chemical science is how molecules originated and how molecules evolved from diatomics to complex ones and then to life itself through self-replicating molecules. The discovery of clathrate hydrates, which are molecular cages of water with trapped atoms or molecules, in interstellar medium suggests that chemistry can happen in their cages leading to more complex molecules. Discovering new chemistry in such cages will be a new direction in molecular evolution.
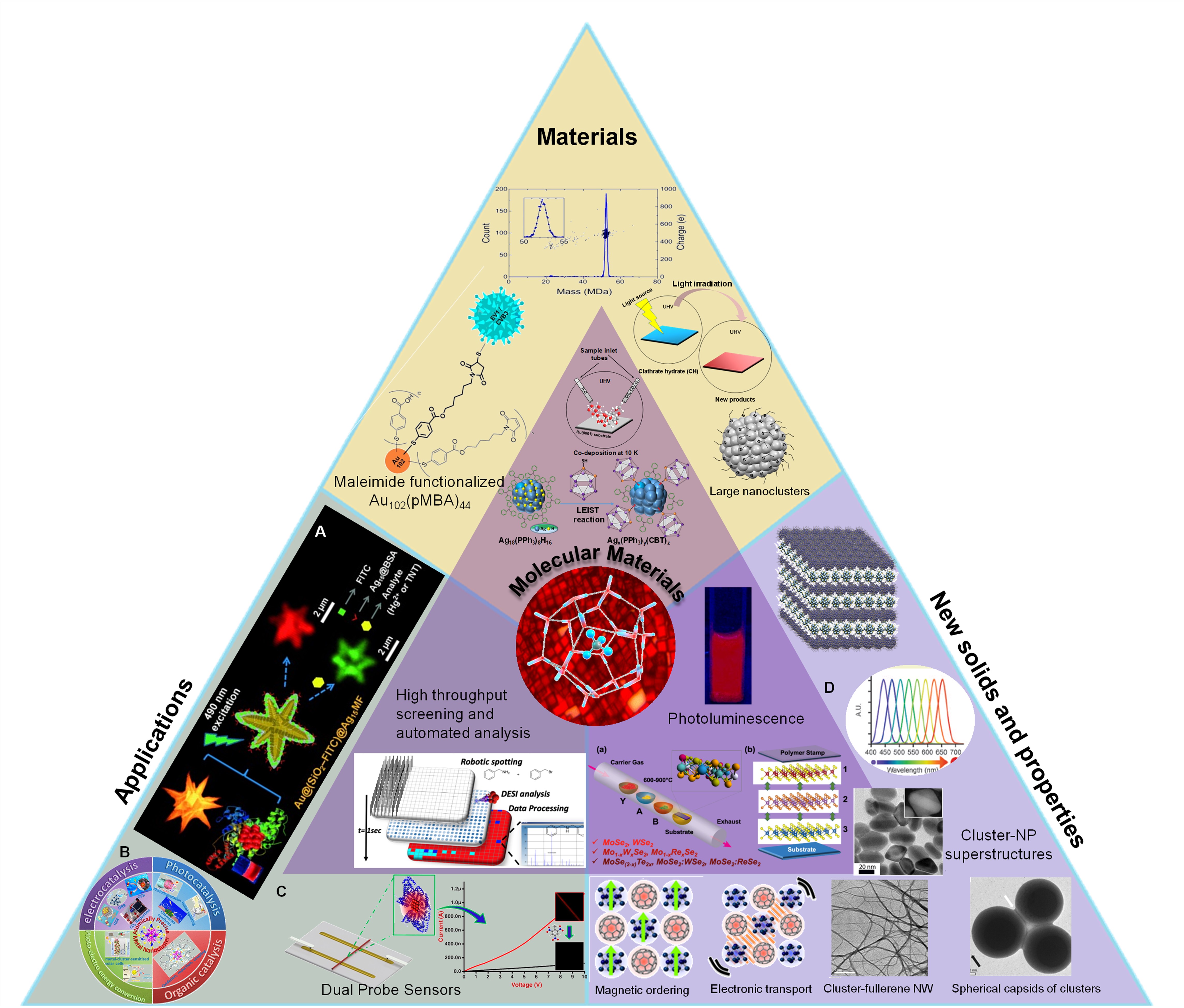
Phase wise planning of the various research projects captured in the figure. Some of the plans outlined in the proposal are not presented here. Inner and outer triangle is for phase I and II, respectively. (A), (C) are taken from PI’s paper, (B) and (D) adapted from Du, Y.; Sheng, H.; Astruc, D.; Zhu, M., Chem. Rev. 2020, 120, 526-622 and Claridge, S. A.; Castleman, A. W.; Khanna, S. N.; Murray, C. B.; Sen, A.; Weiss, P. S., ACS Nano 2009, 3 , 244-255, respectively.
Most of the atomically precise systems known today behave like molecular entities. Their properties are drastically different from the so called plasmonic nanoparticle counterparts. Therefore, a fundamental question would be – where exactly would this transition occur? There have been numerous efforts in this direction already through size-controlled synthesis of nanoparticles. Current understandings point to the fact that it requires studying atomically precise systems having core atoms of a few hundred to thousand atoms. For understanding this phenomenon in detail, such systems need to be synthesized, assembled as solids and characterized in detail. We will outline various methods we are planning to achieve this.
Atomically precise clusters from kDa to Mda
-
New Methods: Proposed synthetic methodologies for creating MDa nanoclusters (NCs) are shown in the scheme below:
-
Separation and purification: In the proposed project, several synthetic methods controlling the growth of nanoparticle nuclei will be developed followed by specific methods of separation resulting in larger clusters.
-
Understanding molecular building blocks: performing mass spectrometry in higher mass range.
- Adaption of a modified commercial QToF instrument to characterize metal clusters and clusters of clusters will be an early goal of this project. Such mass spectral capabilities can achieve the analysis of naked particles with 25000 gold atoms or protected particles 15000 gold atoms with appropriate ligands.
Figure 1. Accurate mass measurement above 1 mega Dalton using attachment of zero (orange), one (green) and two (blue) deca-anions to label E coli ribosome particles 30S, 50S and 70S using a modified QToF mass spectrometer. Abdi Abdillahi, Kenny Lee, Anthony Pitts-McCoy, Scott McLuckey, Purdue University, unpublished.
Chemistry and physics of isolated clusters and cluster ions
-
Chemistry in solution: We propose to conduct intercluster reactions with various transition metal nanomaterials, oxides and chalcogenides to create hitherto unknown clusters. This is particularly interesting as intercluster reactions have shown the possibility to create clusters which have been difficult by direct reactions such as Ir3Au22(PET)18.
-
Chemistry in gas phase: We wish to explore gas phase science of MDa cluster ions. This would include isomerism, dissociation and dynamics, although some of these changes could be too subtle to be explored in these systems, along the lines of our previous work.1 Methods of ion mobility mass spectrometry will be used in this regard. Cluster ions are expected to undergo interesting structural alterations, which could involve the motions of both core atoms and surface ligands.
-
Spectroscopy in the gas phase: Intense ion beams of nA current may be possible to MDa clusters. We are in the process of building such a capability. This will allow the examination of gas phase spectroscopy, especially photoelectron spectroscopy (PES) of MDa cluster anions.
-
Thermally and chemically stable cluster materials: Thermal and chemical stability of clusters depend on ligands and ligand-cluster binding. Our research has shown that carborane-functionalised clusters exhibit high thermal stability. Due to their small size, such ligands can be incorporated on surfaces of large clusters. This work will depend upon the capacity to make a diverse category of carboranes and their functionalized entities.
Microdroplet chemistry for new materials
-
Microdroplet synthesis and diversity: Our objective will be to use high throughput microdroplet synthesis and analysis to rapidly synthesize, deposit, and characterize a variety of new molecular materials.
Figure 2. A demonstration how desorption electrospray ionization (DESI) mass spectrometry of droplet samples can be used for rapid analysis of large volumes of samples. Robots are used to spot samples on a moving substrate and DESI is used to sample the droplets. DESI is an ionization method, introduced by Cooks. The experiment takes <1 sec per reaction well, with data obtained on ~ 6,000 reactions/hour.
Assemblies and properties
-
Single crystal diffraction and related measurements: When crystals are at smaller length scale, it is necessary to use electron diffraction to understand structures. This method is also available for isolated metal cluster ions. Low-dose electron microscopy has also become extremely useful in determining structures of clusters.
-
Cluster co-crystals: In the recent past, a particularly important family of materials have emerged which are called cluster co-crystals. The similarity of clusters in size and shape makes it possible to create assemblies of different clusters in the same lattice.
Figure 3. (A) Structural anatomy of the Ag40(SR)24(PPh3)8. (B) Structural anatomy of the Ag46(SR)24(PPh3)8. (C) Packing of Ag40 or Ag46 nanoclusters into a centred rectangular 2D lattice. (D) Encapsulation of Ag40 and Ag46 nanoclusters in a single crystal to create a co-crystal. Adapted from ref. (2) Bodiuzzaman, M. et al. Angewandte Chemie International Edition 2019, 58 (1), 189-194.
-
Cluster assemblies: Supramolecular chemistry is a major area of chemistry that utilizes weaker, non-covalent interactions between molecules. They include hydrogen bonding, van der Waals, electrostatic, π…π and C-H…π interactions. Such forces have been the basis of several molecular self-assemblies and host-guest complexes in organic, inorganic and biological systems.
Figure 4. Visualizing the diverse possibilities in cluster assemblies. A) Structures of clusters (modelled from co-ordinates of crystal structures) which can act as the starting materials.3,4-8 B) different types of assemblies formed with clusters,9-12 C) TEM images of such cluster-assembled superstructures,9,13-14 and D) schematic showing a cluster-assembled material, which could be probed by a variety of tools.
-
Nanoclusters as next-generation combustion fuels: The hypergolic activity of the nanoclusters with varying ignition time. It may be noted that these nanoclusters are remarkably inert towards temperature, friction, impact and electrostatic discharge, ensuring safety in their handling and storage. There is a possibility of synthesizing several other carborane based nanoclusters with varying ligand functionality and metallic core for such purposes.
Figure 5. Photographic images of the ignition process of o-carborane, HC≡C-CBA, Ag14-CBA and Cu6Ag8-CBA in presence of fuming nitric acid as an oxidizer. No hypergolic activity was observed for o-carborane and HC≡C-CBA molecules. But both the nanoclusters showed hypergolic activity with varying sensitivity. (Adapted from Ref. 15) Wang, Q.-Y. et al. Journal of the American Chemical Society 2020, 142 (28), 12010-12014.
-
Creating defect-free materials: Atom probe tomography and associated methods: Atom Probe Tomography (APT) is a versatile technique for material characterization providing information about three-dimensional structure of the sample as well as its chemical composition. Its capability to provide information at atomic scale resolution (around 0.1-0.3 nm resolution in depth and 0.3-0.5 nm laterally) makes it relevant in the context of understanding atomically precise cluster assembled solids.
Figure 6. Shows the data collected from a single crystal of Ag12(TBT)6(pyz)6(TFA)6(CH3CN)2 (abbreviated as Ag12-MOF). (a) Optical image of single crystals of Ag12-MOF. (b) Ball-and-stick model of Ag12-MOF showing a layered structure. (c and d) FESEM image of (c) single crystals of Ag12-MOF and (d) a needle prepared from the single crystal of Ag12-MOF using focused ion beam milling. (e) 3D reconstruction of an APT needle. (f) High-resolution atom maps showing the atomistic distribution within the single crystal of Ag12-MOF. The layered structure is evident. (Adapted from Ref 16) Ananthu Mahendranath et al., unpublished.
-
Computational studies: A range of computational methods will be used for the work planned in the Centre. For certain problems, we will use classical methods. For example, we will carry out structural optimizations, Monte Carlo calculations and molecular dynamics simulations using interatomic force fields.17,18 Properties such as the stability of a material, its response to external fields, and interfaces with other materials and molecules are governed by the nature of interaction between atoms mediated by electrons. These interatomic interactions are accurately captured by first-principles density functional theory (DFT) calculations.19,20 We will use state-of-the-art DFT calculations in the prediction of the structure of nanoclusters, as well as in their interaction with the 2D materials of relevance to the development of composites, and molecular intermediates that are relevant to the role of clusters in catalyzing important chemical reactions. Once a database of DFT results with information about electronic structure and atomistic details has been established, we will determine descriptors (easily computable features that correlate with a property of interest) of their properties, such as structure, electronic and mechanical properties, and catalytic activity. The use of high throughput calculations and descriptors in rational materials design has been reviewed in a recent book chapter.20 We plan to use feature selection algorithms of machine learning21 to identify optimal descriptors of properties such as catalytic activity, magnetism and superconductivity, using a combination of experimental and DFT-derived data.
-
Cryo-chemistry in hydrate cages: Research on gas hydrates will be built on our finding that they can exist in cryogenic and low-pressure conditions as in space. As over 240+ molecular entities are present in the ISM, it is likely that many different gas hydrates are present in such an environment, although at low concentrations. Along with ultraviolet photons, other high energy radiations are preset in such environments and interesting photochemistry is possible in the cages of CHs. We plan to simulate conditions of relevance in the laboratory. To conduct such studies, we have built an ultra-high vacuum low energy ion scattering spectrometer (Figure 7) which houses several facilities such as reflection absorption infrared (RAIR) spectroscopy, secondary ion mass spectrometry (SIMS) and temperature programmed desorption (TPD).
Figure 7. Photograph of the state-of-art instrument consisting of a UHV chamber. It has a Ru(0001) substrate on which molecular solids are grown at 10 K. The experimental chamber is combined with different spectroscopic tools for analysis. These tools are: (1) Reflection absorption infrared spectroscopy (RAIRS), (2) Temperature programmed desorption-mass spectrometry (TPD-MS), (3) Low energy ion scattering-mass spectrometry (LEIS-MS), and (4) Secondary ion mass spectrometry (SIMS).
Expected deliverables of the research
Phase 1: (Year 1-2)
- Synthetic methods for controlled growth of nanoparticle nuclei with and without monolayer protection.
- New cluster compounds and their 2D and 3D assemblies.
- Creating molecular materials through microdroplet synthesis.
- Defect-free atomically precise materials and elucidation of their atomistic details through electron tomographic reconstruction, APT, and XRD.
- Cocrystals of different clusters leading to composite properties.
- High-flux electrospray to produce cluster ion beams and spectroscopy of such ions.
- New chemical reactions within the cages of clathrates and understanding elementary processes in them.
- Detailed understanding of the properties through methods of computational materials science.
Target Number of Papers: 20, 5 patents
Phase 2: (Year 3-5)
- Development of technique like Mega-Dalton Mass Spectrometry to enhance the understanding of nanomaterials at molecular level.
- Synthesis of giant nanoclusters and characterization by high resolution single crystal X-ray diffraction and Mega-Dalton mass spectrometry.
- High-end structural characterisation, such as cryo-electron microscopy and analysis of linkage of nanocluster-bio molecules.
- Exploring exotic properties like electro, phonon transport properties, photoconductivity, magnetism and superconductivity.
- Applications in sensing and catalysis including electroreduction of CO2.
Target Number of Papers: 80, 15 patents
Societal impact
New materials will be utilized in several ways by international research groups involved with catalysis, CO2 reduction, water splitting and so on. Mass spectrometric methods will be used to understand the respective elementary reactions. Gold, silver, palladium and platinum clusters supported on different surfaces such as TiO2, ZrO2, and CeO2 have already been used for catalysis, CO oxidation, water splitting, and solar cells. Other applications of this type of clusters are in solar cells, replacing the dye. Glutathione protected gold clusters have been used for water-splitting.
Sustenance statement
With industrial collaborations and linkages, we expect to have one new project in Phase I and at least two in Phase II. One new sponsored project in Phase I and two in Phase II are expected. Annual funding to the tune of Rs. 2 crores among all the faculty is expected at the end of five years, from national and international agencies. This will be adequate to run the centre with essential activities. The programs such as winter school, conferences, international exchange, etc., will attract alumni funds for their sustenance.