Project
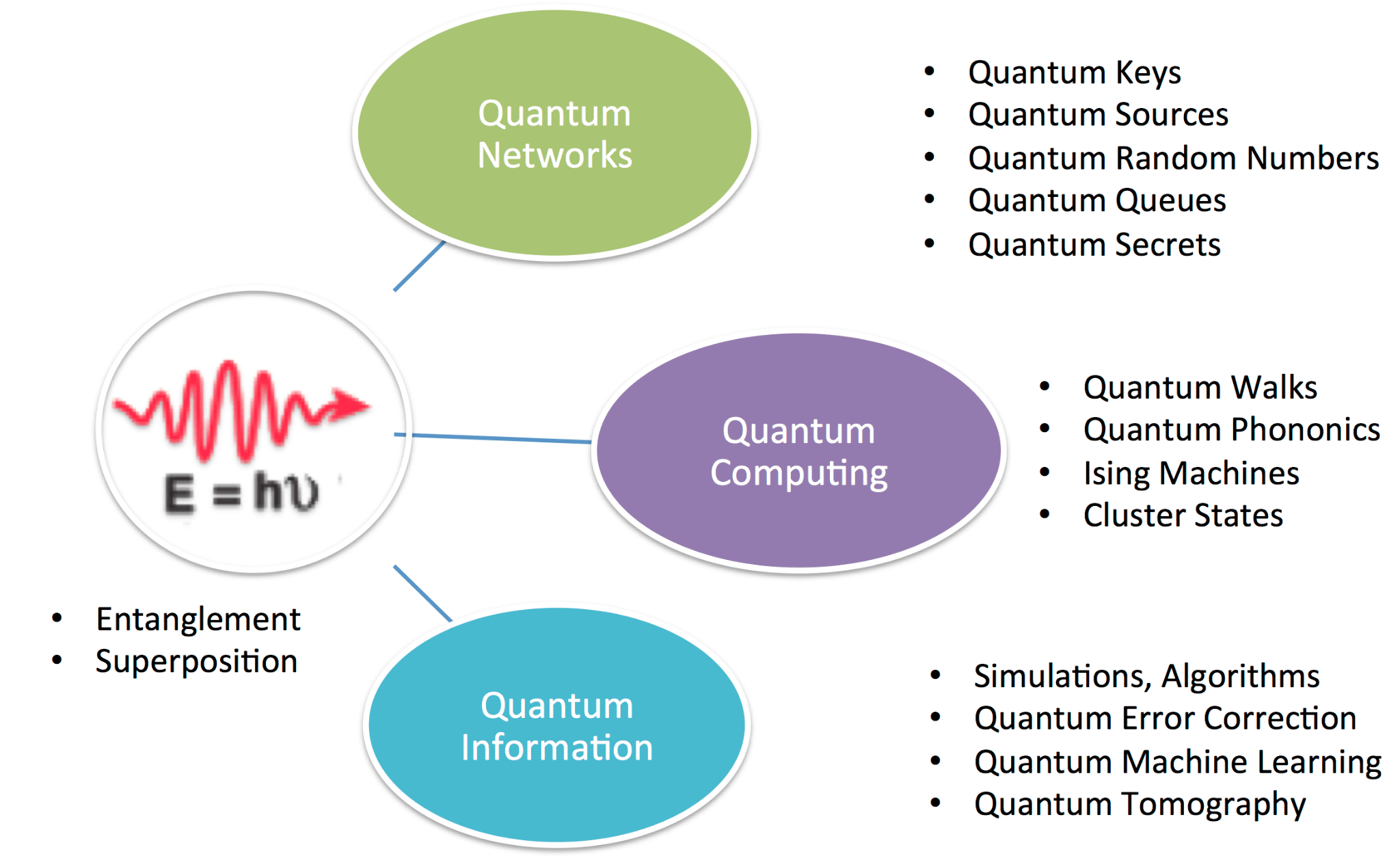
Focus Areas of Research
India’s first Metro Area Quantum Access Network (MAQAN), is proposed between IITM, IITMRP, SETS and NIC. This is conceived as a testbed with multiple short haul link, to explore implementations of quantum communication and develop standards around them. Indigenously built hardware for differential phase reference quantum key distribution will be extended to other QKD protocols such as measurement device independent QKD, continuous variable QKD and other communication paradigms such as secret sharing.
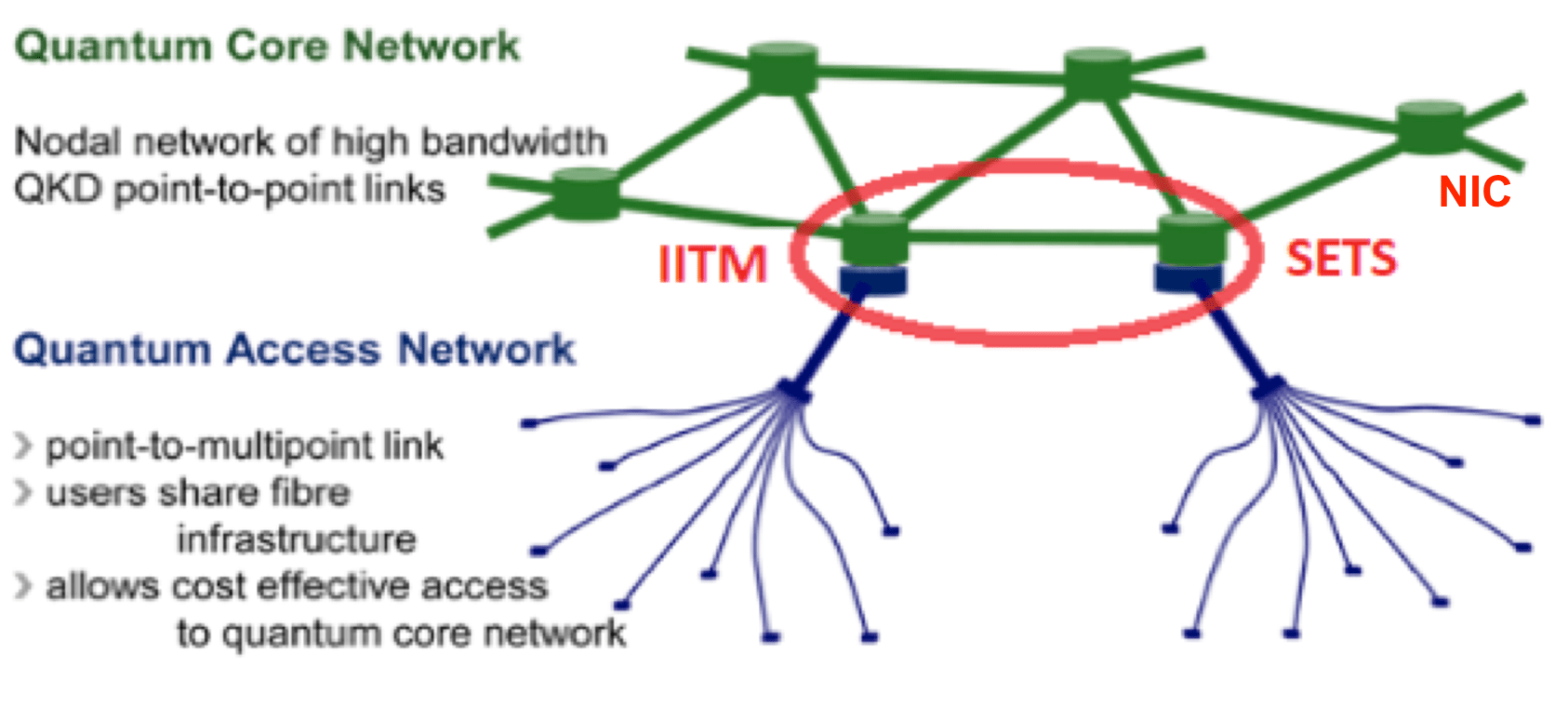
Metro Area Quantum Access Network
The growing access to devices on the quantum cloud enable a variety of use cases from basic science to translational research and industrial applications. The members of QuICC undertake research on quantum algorithms, simulations and interdisciplinary applications that make use of constraint optimization, machine learning with domain knowledge ranging from chemistry to finance to cryptography.
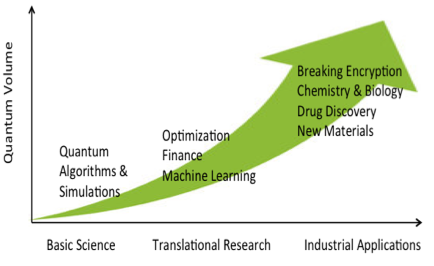
Growing interest in quantum computing
The Centre on Quantum Information, Communication and Computing brings together the interdisciplinary expertise in IIT Madras across the disciplines of Engineering, Science and Management to tackle some of the key scientific and technological challenges in this frontier research area. The focus areas of the centre can be organized under two broad themes of (1) Quantum Networks, and (2) Quantum Computing and Simulations. On these topics we will be addressing problems at every level, starting from theoretical aspects all the way up to devices and systems. The Quantum Internet conceptualizes secure communication where the security is based on the laws of quantum physics. In this proposal we address some of the key challenges in building a metro area quantum access network, such as identifying optimal scheduling and routing protocols. Also, we will be developing protocols for entanglement sharing and quantum key distribution (QKD). Finally, we will standardize the communication equipment for deployment and scaling of the network ensuring a high quality of service with security. Another important component of our proposal is dedicated to making IIT Madras an IBM quantum hub. A membership in IBM Q network will allow access for the 53-qubit IBM quantum processor and currently there are only 34 academic institutions with membership. This will be the first IBM Q hub in India and would attract academic and industry partners and thus play a major role in developing the quantum ecosystem in India.
Work Plan
The research work at the Centre of Excellence (CoE) for Quantum Information, Communication and Computing, falls under two broad areas of Quantum Networks and Quantum Computing. Each of these areas requires an understanding of basic and applied science, design engineering and instrumentation and metrology making it highly interdisciplinary. The key technology platforms for development are illustrated in Figure 1 below.
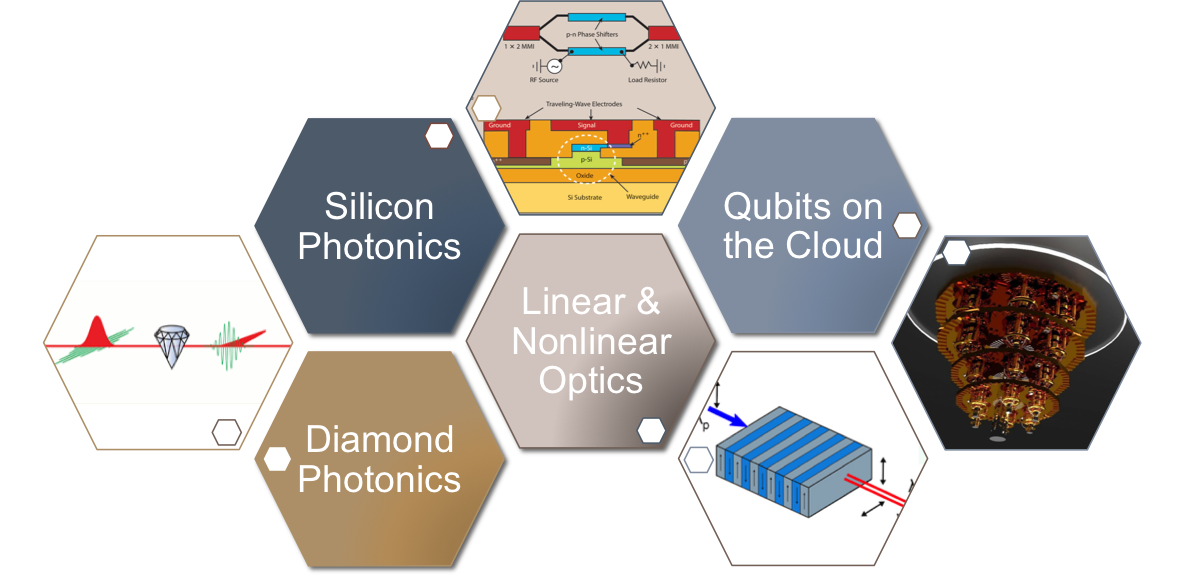
Figure 1: Key technology platforms at CQuICC
MAQAN: Metro Area Quantum Access Networks
Development of a quantum network using the fiber optic backbone in IIT Madras and it connection to IITM Research Park and extend it to the Society for Electronic Transactions Security (SETS). Using this quantum network we can test quantum communications and also demonstrate different protocols used in quantum communication. We can do this by understanding the basic point to multipoint QKD networks. The network will be wavelength multiplexed with multiple transmitters and a single superconducting nanowire single photon detector (SNSPD), shown schematically in Figure 2
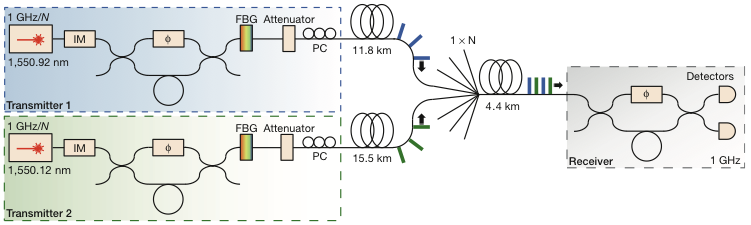
Figure 2: Point to multipoint architecture [from Frolich et al, Nature, 2013]
Entangled photon source
Photonic qubits are one of the important types of qubits and are the usual means to transfer quantum information. Entangled photonic qubits form the basis for quantum information systems. At CQuICC, we will develop entangled photonic source and cluster states for use in quantum communication and computation. Using spontaneous parametric down conversion (SPDC) we will develop entangled photon sources and then we will quantify these sources using the Hong-Ou-Mandel interferometer [HOM87]. Some of the important tests are the demonstration of entanglement using CHSH inequality [CHSH70] and the second order photon correlation to characterize the photon. An experimental setup for the second order photon correlation and the measured signals are given in Figure 3 below.
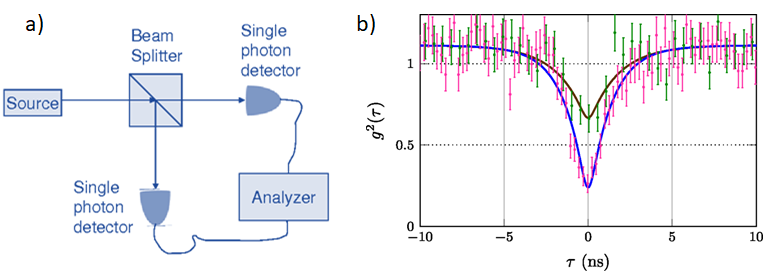
Figure 3: (a) The experimental setup for the second-order correlation of photons. (b) The signals from a typical second order correlation.
Optical Comb Sources
The development of large scale photonic integrated circuits for precisely controllable optical functions to enable practical nonclassical secured communications, metrology and meaningful quantum simulation and computation is being proposed. A microring resonator (MRR) in a silicon photonics platform is a potential candidate and recently we have demonstrated silicon MRR [KRL+ 19, FB19] characterizing loaded Q-value as high as 105 with free spectral range (FSR) as low as 10 GHz, using the silicon photonics technology developed at IIT Madras. We also plan to integrate the comb source into photonic integrated circuits and demonstration of quantum photonic functions.
Quantum Random Number Generators (QRNGs)
Two quantum random number generators have been demonstrated using weak coherent sources at 1550 nm. Through our work we plan to miniaturize one of them, viz the photonic lantern quantum random number generator (PL-QNRG) using a femtosecond laser to modify the refractive index of a glass chip. A schematic diagram of the device with a mode selective PL and a non-mode selective PL are shown in Figure 4 below. A successful demonstration of the device has already been done [PS20] and we are currently in the process of fabricating this on a mm-size chip and package it.
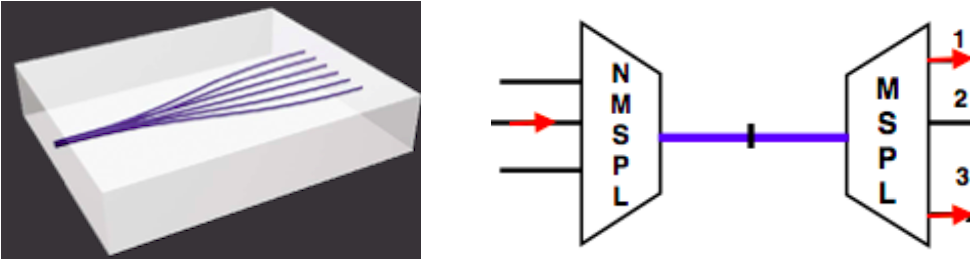
Figure 4: Schematic diagram of Photonic Latern and the structure needed for PL-QRNG
MASER
MASER is a microwave equivalent of LASER. It has been very challenging to develop a scalable Maser technology that can work at room temperature. Recently [BSS+ 18] it has been reported that NV-centers in diamonds can be potential candidates for the development of MASER. At the Centre of Excellence we aim to demonstrate Masing from diamond samples and use it in quantum technology oriented applications.
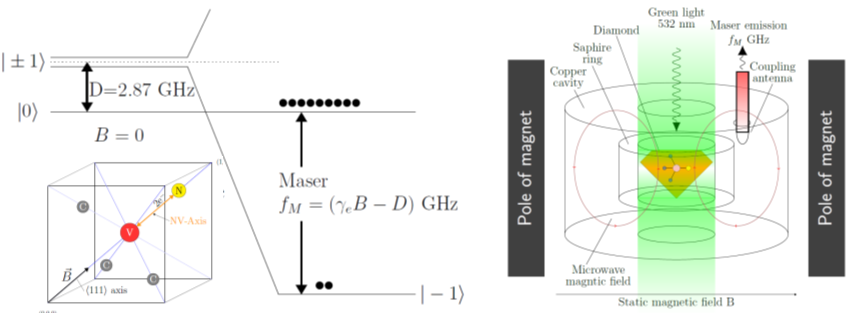
Figure 5: (Left) Energy level diagram of NV center for population inversion and masing. (Right) Schematic sketch of a Maser experiment from Ref. [BSS+ 18].
Queuing, Scheduling and Quantum Queue-Channels:
A Queue is a setting where a stream of qubits is processed sequentially. Modelling the sequential processing of qubits using a single server queue and calculating the classical capacity of the quantum queue channel has already been done. This work opens up a whole new set of problems involving scheduling and load balancing for serial parallel quantum information processing tasks with decohering qubits. In future work, we propose to model and analyze important QIP tasks like the quantum Fourier transform with decohering qubits through the quantum queuing lens.
Quantum Secret Sharing:
Cryptography is one of the earliest applications of quantum information processing. It is much more secure than classical cryptography and this enhanced security is a consequence of the properties of quantum information. At the Centre of Excellence we propose to develop a comprehensive theory of communication efficient secret sharing schemes. This study will help us to go beyond QKD to study quantum cryptography. We would then like to optimize the secret sharing schemes for quantum technologies. In particular we plan to develop efficient secret sharing schemes for the NV center based qubits and photonic qubits. We can also implement secret sharing schemes over the established access network testbed.
Quantum Sources:
A cluster state is a giant, highly entangled “quantum resource” state in which all qubits in the calculation have been interconnected in advance. These states are used in one-way quantum computing which proceeds in a series of local, single-site measurements in a specific order and quantum basis. The outcome of each step is logged classically and becomes the input for the next step. In general, a quantum algorithm is encoded in a pattern of individual measurements and the classical data from the individual quantum measurements gives the program’s final result. The initial cluster is destroyed in the course of the computation. Another source is the squeezing comb source in which we will build optical parametric oscillator (OPO) cavities that exhibit comb lines with noise at least 6 dB below the quantum noise level. The squeezing combs find applications in a number of quantum imaging experiments, with potential uses in secret sharing. This will form the building blocks for cluster states and photonic quantum computing [LGB+ 19].
Quantum Phononics
Quantum phononics is a new field in which phonon modes are used for communication and computation. This requires the ability to maintain phonon modes for long times before the modes get damped and also the ability of the mode to interact with electric, magnetic and optical systems. Current advances in the field requires state-of-the-art nanofabrication and refrigeration capabilities, making them impracticable for scalable practical deployment. The goal here will be to design phonon emission devices, resonator systems and detection methods.
Tomograms, information and nonclassical effects
A tomogram is a set of histogram of judiciously chosen observables. Quantum state can be reconstructed from tomogram. The problem gets accentuated if the Hilbert space is large as in the case of quantum optics, systems comprising many qubits. This poses major challenges even today in the context of identification of new nonclassical stats in the laboratory. It is important to extract as much information on nonclassical effects such as quantum entanglement and squeezing properties directly from tomograms. The aim of our project is devise ways to extract the indicators of entanglement directly from the tomogram and estimate its efficiency by comparing it with standard measures of entanglement like subsystem von Neumann entropy which can be obtained from the reconstructed state. Our objectives in this regard will be two-fold namely (a) identify multipartite entanglement indicators directly from tomograms, and (b) examine the role of both bipartite and multipartite entanglement in multipartite continuous variable and hybrid quantum systems using IBM Q platform.
Cloud based Quantum computing and Simulations
Cloud based quantum computing is a novel idea which provides access to remote quantum computers where the various protocols and algorithms can be explored. It can also help in the development of new algorithms which may otherwise appear to be too abstract for the classical supercomputer. A recent attempt by Google Quantum AI at demonstrating so-called quantum supremacy was in this direction [AAB+ 19]. These simulators can implement quantum circuits, add noise to the circuits and also help to verify protocols. In a classical computer we cannot simulate more than 18 qubits exactly as the relevant Hilbert space of states grows exponentially with particle number. Many special techniques like Density Matrix Renormalization Group (DMRG), Matrix Product States, and Symmetry reductions, have been evolved for this purpose and help to some extent. These methods also have their own restrictions and hence we can use cloud based quantum simulators and quantum computers to go beyond these restrictions. As a variety of quantum hardware is being developed, it is also essential to develop algorithms and quantum circuits that can solve real problems better than the best classical computers can.
The IBM Quantum Experience which has been on the cloud since 2016 has already attracted hundreds of thousands of users who are building libraries of specialized quantum circuits, even with architectures having as few as 5 qubits. From a pedagogical perspective, with electives such as Quantum Information and Computation and the new Dual Degree program in Quantum Science and Technology being offered in IIT Madras, such a resource is invaluable, one may even argue essential, in training students. From a research perspective, this will enable us to implement various protocols on it and study a range of problems. For instance, it is only in the last few years that a time-evolving non-stationary quantum state has been “kept alive” for a significant amount of time, in the sense that it largely remains pure uncontaminated by environmental noise. Some applications of using the IBM Quantum computer would be in (a) Quantum Error Correction (QEC) and fault tolerance in NISQ devices and (b) Information Scrambling and quantum random circuits.
Many-body localization on IBM Q and quantum memories
A study of the few and many-body systems like kicked tops, and many-body integrable and non-integrable spin chains will be done using IBMQ. To study a many body system with typical interactions it is essential to understand the role of nonintegrability so as to control the consequences. This will require understanding entanglement and nonclassical correlations in the context of thermalization and localization. The transition from ergodic and thermal phases to potentially localized phases can prove to be of importance to quantum registers. There is a preliminary evidence that even in “clean” systems without disorder there can be localized phase depending on the relative strengths of interaction and one-body potentials. We can use a quantum computer to study such MBL. We propose to use quantum machines such as the devices on the cloud as possible route to overcome this issue and demonstrate an ergodic to localized transition. A related aspect is to study possible MBL phases in higher dimensional systems, as almost all current studies are one-dimensional.
Quantum Machine Learning
Quantum Unconstrained Boundary Optimization problems are NP complete problems and some of these problems can be solved on quantum machines. Any new quantum hardware can be benchmarked using a QUBO problem. Once that is done, attempts can be made to push other Machine learning techniques and algorithms for quantum computing. We will set up the mathematical foundations to convert a complex optimization problem as QUBO and solve these on quantum devices. Test the precision of the mathematical methods and check for optimality. Set up a performance matrix to evaluate a design quantum machine using QUBO as a base. Ising machines solve QUBOs by minimizing the energy associated in a lattice of coupled spins. We will build and demonstrate the ability to solve a few QUBOs on coherent Ising machines, and understand the issues related to scaling up of the photonic platforms through other annealers available on the cloud.
Quantum multi-arm bandits and Quantum reinforcement learning (RL)
The Bandit learning method involves identifying the best option from a given set of data and these options are referred to as arms. We look into only the statistical samples that are obtained by playing each arm. The bandit arms can be viewed as quantum registers [DTB16, DLWT17] and have shown a quadratic speed-up in best arm identification. Some interesting problems are identifying the arm with the underlying density operator with the highest von-Neumann entropy or the best purity and so on. This would require deriving or adapting concentration inequalities for density operators. We also aim to tackle quantum Reinforcement Learning (RL) problems and investigate the learning performance of agents quantum accessible RL environments.
References
-
[AAB+19] Frank Arute, Kunal Arya, Ryan Babbush, Dave Bacon, Joseph C Bardin, Rami Barends, Rupak Biswas, Sergio Boixo, Fernando GSL Brandao, David A Buell, et al. Quantum supremacy using a programmable superconducting processor
Nature, 574(7779):505–510, 2019. -
[BSS+18] J D Breeze, E Salvadori, J Sathian, N M Alford, and C W M Kay Continuous-wave room temperature diamond maser Nature, 555:493–496, 2018.
-
[CHSH70] John F Clauser, Michael A Horne, Abner Shimony, and Richard A Holt Proposed experiment to test local hidden variable theories Physical Review Letters, 24(10):549, 1970.
-
[DTB16] Vedran Dunjko, Jacob M Taylor, and Hans J Briegel Quantum-enhanced machine learning Physical review letters, 117(13):130501, 2016.
-
[DLWT17] Vedran Dunjko, Yi-Kai Liu, Xingyao Wu, and Jacob M Taylor Exponential improvements for quantum-accessible reinforcement learning arXiv preprint arXiv:1710.11160, 2017.
-
[FB19] Tara Fortier and Esther Baumann 20 years of developments in optical frequency comb technology and applications Communications Physics, 2(1):1–16, 2019.
-
[HOM87] Chong-Ki Hong, Zhe-Yu Ou, and Leonard Mandel. Measurement of subpicosecond time intervals between two photons by interference. Physical Review Letters, 59(18):2044, 1987.
-
[JMZL17] Bhargavi Jonnadula, Prabha Mandayam, Karol Zyczkowski, and Arul Lakshminarayan. Impact of local dynamics on entangling power Physical Review A, 95(4):040302, 2017.
-
[MJC20] P. Mandayam, K Jagannathan, and A. Chatterjee. The classical capacity of additive quantum queue-channels. IEEE Journal on Selected Areas in Information Theory (Special issue on Quantum Information Science), 1, 2020.
-
[LGB+19] Mikkel V. Larsen, Xueshi Guo, Casper R. Breum, Jonas S. Neergaard-Nielsen, and Ulrik L. Andersen. Deterministic generation of a two-dimensional cluster state Science, 366(6463):369–372, 2019.
-
[Pre18] John Preskill. Quantum computing in the nisq era and beyond. Quantum, 2:79, 2018.
-
[PS20] Anil Prabhakar and Gautam Shaw. Photonic lanterns for quantum random number generation, Sept. 2020.
-
[Q+ 20] Google AI Quantum et al. Hartree-fock on a superconducting qubit quantum computer. Science, 369(6507):1084–1089, 2020.
-
[RPM19] Shashank Kumar Ranu, Anil Prabhakar, and Prabha Mandayam. Differential phase encoded measurement-device-independent quantum key distribution. arXiv preprint arXiv:1905.11153, 2019.
-
[SLB20b] B Sharmila, S Lakshmibala, and V Balakrishnan. Tomographic entanglement indicators in multipartite systems. Quantum Information Processing, 19(4):1–12, 2020.
-
[SSLB17] B Sharmila, K Saumitran, S Lakshmibala, and V Balakrishnan. Signatures of nonclassical effects in optical tomograms. Journal of Physics B: Atomic, Molecular and Optical Physics, 50(4):045501, 2017.
-
[SSR+20] Gautam Shaw, Shyam Sridharan, Shashank Ranu, Foram Shingala, Prabha Mandayam and Anil Prabhakar. Equivalence of space and time-bins in DPS-QKD. arXiv preprint arXiv:2008.03083, 2020.
-
[ZZD+16] Quntao Zhuang, Zheshen Zhang, Justin Dove, Franco NC Wong, and Jeffrey H Shapiro. Floodlight quantum key distribution: A practical route to gigabit-per-second secret-key rates. Physical Review A, 94(1):012322, 2016.
Expected deliverables of the research
Phase I
Quantum Networks
-
The development of a network of physically separated quantum systems capable of exchanging quantum information between themselves.
-
Demonstration of entanglement sharing and transfer between different nodes of the network.
-
Characterization of delays and noise in a network of quantum systems and an analysis of the quantum vector cost analysis of the network.
-
Based on the quantum vector cost analysis the development of optimal scheduling and routing protocols for quantum networks.
-
Investigating the atmospheric effects on the quantum communication between different nodes of a network using quantum states carried by drones.
Quantum Computing and Simulation:
-
Development of a small and portable Quantum Random Number Generator (QNRG)
-
Creating a source of entangled photons based on the principles of Spontaneous parametric down conversion (SPDC).
-
Development of the four qubit quantum error correcting codes and benchmarking their Performance through the unprotected single qubit.
-
Creation of Spatial and temporal Ising model and using it solve Quantum Unconstrained Boundary Optimization (QUBO) problems which has applications in Machine Learning, Finance and economics
-
Experimental demonstration of Quantum Key Distribution (QKD) protocols using Qudits.
-
Design of Quantum Random Circuits with a view to study entanglement spreading and Information scrambling.
-
Implementation of Quantum state transfer protocols and application of error correction to improve the fidelity of such protocols.
-
To use Quantum reinforcement learning for Quantum optimization routines.
-
New techniques for entanglement detection and measurement directly using tomograms.
Phase II
Development of new quantum technologies
-
Development of a Metro Area Quantum Access Network and secret sharing over this network and also carrying out a security analysis of the network.
-
Comparative analysis of QKD and Secret Sharing protocols for transfer of quantum information transfer with maximum secure finite key rates
-
Experimental development of optical frequency comb, photonic cluster states and generating their tomograms.
-
Quantum phononics based experiments for testing different quantum information and communication protocols. Quantum photonics based annealer with remote access to solve Quantum Unconstrained Boundary Optimization (QUBO) problems.
-
Experimental generation of 2D cluster states and using it for one-way quantum computation.
-
Introduction and testing of multiprtite tomogram based Entanglement detection and measurement and verifying it with experimental results as well as numerical simulation on the IBM quantum experience platform.
-
Design of experimental devices like MASER, Optical Comb and Single quantum emitters
Current status
- Ising Machines that can solve MAXCUT and NP-Hard problems winners of the 2020 Tayur Prize, Feb’21
- Kickoff meeting of MAQAN, Apr’21
Collaborations
International Collaborations
- Prof. David DiVincenzo, RWTH, Aachen and Julich
- Prof. Sridhar Tayur, Carnegie Mellon University, Pittsburgh
- Prof. Hyunsoo Yang, NUS, Singapore
- Prof. Hui Khoon Ng, Yale-NUS College, CQT, Singapore
- Prof. Sridhar Tayur, CMU, Pittsburgh, USA
- Prof. Michele Heurs, Leibniz University, Hannover, Germany
- Prof. J ̈org Schulze, Univ. of Stuttgart, Germany
- Prof. Surendra P. Singh, Univ. of Arkansas, USA
- Prof. Daniel Stancil, NCSU, Raleigh, USA
Industrial collaborations
- KLA - India
- Bharat Electronics - Corporate Research Lab
External international site links for project
International education programs
- Introduction to Quantum Computing, Centre for Continuing Education, May’21
- IITM - RWTH workshop : June’21
Societal impact
Quantum technologies will play a key role in reshaping our future. Quantum secure communications become a necessity in the age of cyberattacks, while quantum computing will enable new applications in finance, chemistry and operations research. Our focus on information, communications and computing will have a multiplicative and disruptive effect, and will address the societal needs of the 21st century.
Sustenance statement
A National Mission on Quantum Technology and Applications has been proposed with the 4 pillars as quantum computing, quantum communications, quantum sensing and quantum materials. The efforts at QuICC are receiving support from different ministries such as MeitY, DST, MHRD and MoD of the Government of India. Further, there is interest from the early adopters of industry who are seeking to leverage off our expertise in quantum machine learning